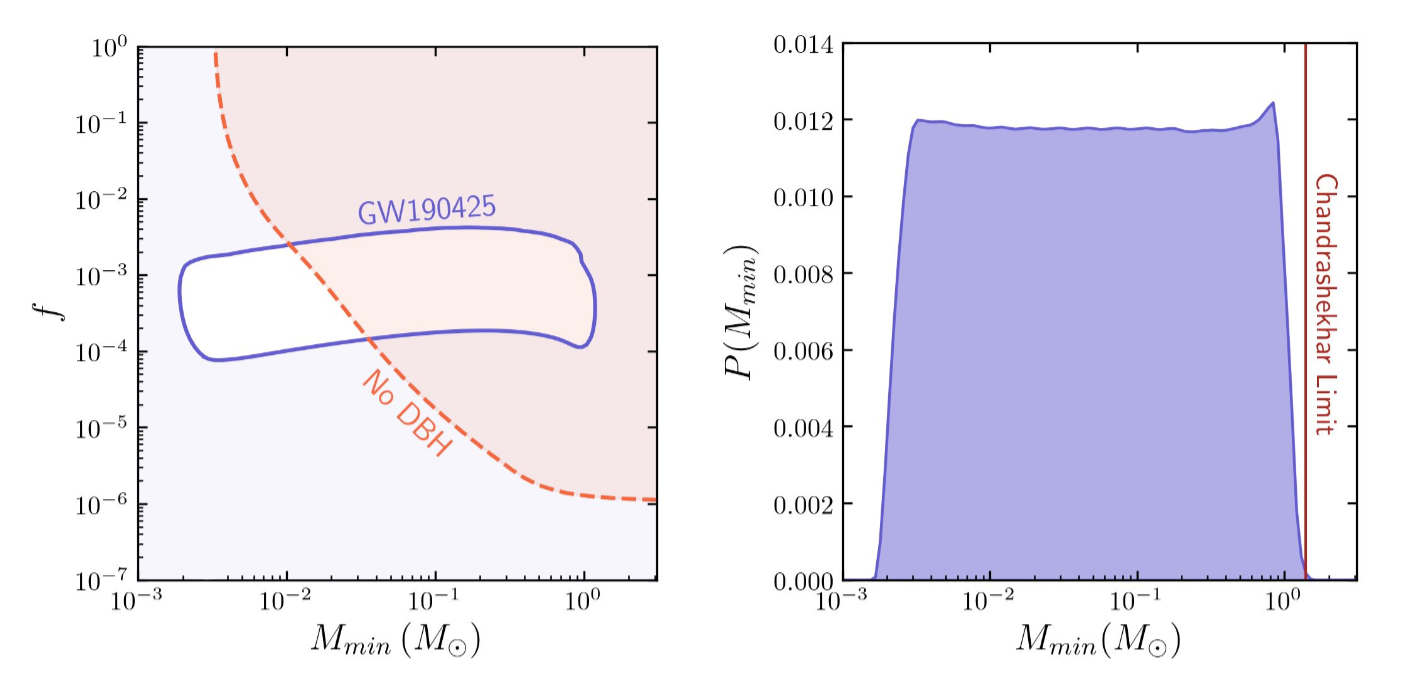
Collaborations
| LIGO |
In the prevailing paradigm, 'cold dark matter' is comprised of a single particle with a weak, non-gravitational interaction with visible matter. This paradigm is increasingly in question as laboratory searches come up empty-handed and observations of the internal structure of galaxies raise persistent, if inconclusive, concerns about how well the predictions of cold dark matter match the data. We propose a new test of a different dark matter paradigm, enabled by the recent success of the LIGO instruments. We postulate that dark matter is more similar than dissimilar to visible matter in the sense that it contains multiple particle species that interact. Particle interactions allow ~1% of dark matter to dissipate kinetic energy and cool, altering small-scale galaxy properties in the direction suggested by data. In this scenario, we predict that a small fraction of the dark matter cooled sufficiently to form black holes. Our model predicts that even 0.1% of dark matter in dark matter black holes is sufficient to produce a substantial rate of black hole mergers for LIGO within 100 million light years of Earth. Some of those mergers will involve objects lighter than 1 solar mass, providing smoking gun evidence of new physics.
We will conduct a survey of sub-solar mass binary compact objects using LIGO gravitational wave data taken between 2019 and 2021. To conduct the search, we will match the gravitational waveforms predicted from general relativity for black holes between 0.1 and 1.0 times the mass of our Sun using standard LIGO search techniques. Our survey volume will contain enough galaxies that we will be sensitive to mergers even if they occur only once per 100,000 years in a typical galaxy. Simultaneously, we will pursue our theoretical effort with the goal of connecting the merger rate to the dark matter physics. Using both analytical and numerical tools, we will study the evolution of the interacting dark matter from the very early universe through the formation and merger of dark black hole binaries. We will refine the estimates of dark black hole populations first made in in order to provide either lower limits or confidence intervals for the mass of a dark proton and to constrain the strength of the interaction between dark particles.
A sub-solar mass black hole detection would be a breakthrough hinting that dark matter has a complex interacting particle spectrum. Even more, the mass of the black hole would directly constrain the mass of a heavy dark-charged particle through the Chandrasekhar relation. Such a result would open the door to work on dark matter physics comparable to what has gone into constructing our own periodic table, as well as the collider physics that confirmed the fundamental constituents of visible matter. Null results of our search will provide constraints on dark matter models inaccessible by any other means. Understanding the majority of matter in the universe (the dark matter), will revolutionize our understanding of the origin of all structure in the universe and provide insight the cosmic environment that makes our galaxy and solar system possible.